Holistic Management™ Australian Quarterly Newsletter, July 2000, pp. 4-6.
Reprinted in the Stipa Newsletter No. 13, Winter 2000, pp. 8-12; The Australian Farm Journal, October 2000, pp. 90-92 and Grass Clippings November 2000 pp. 3-5.
The Great Salinity Debate: Part I
Controlling the salinisation process
Christine Jones
INTRODUCTION
Water is a great solvent, especially for salts, and most moving water carries a variety of dissolved salts to either an ocean, inland sea, or a salt lake. When water evaporates it leaves much of the salt behind, hence water bodies subject to salt inflows and evaporation become progressively saltier. The world's oceans are the result of a "natural" salinisation process which has been going on for millions of years. Soils are also subject to salinisation when soil water carries dissolved salts close to the surface and then evaporates, thereby concentrating the salt.
High loads of innate, connate and cyclic salts are characteristic of much of the Australian environment. Historical records indicate that there were saline scalds, brackish springs and brackish creeks in many parts of the landscape at the time of European settlement. However, the salinisation process in both rivers and on dry land has rapidly accelerated over the last 200 years. That is, the movement of salt has become more active due to changes in soil hydrology (water balance) since European settlement. To this point we all agree. When it comes to why, and how to fix it, we enter the great salinity debate.
I believe that many incorrect assumptions have been made about dryland salinity, and therefore many ill-conceived and simplistic "solutions" have been proposed. So let's start with what we know. Two hundred years ago, most of the areas currently affected by dryland salinity were open grassy woodlands (widely spaced trees with a grass/forb understorey), grasslands (no trees) or shrublands. Much confusion regarding this pre-European vegetation exists. Due to a complex of interacting factors including the loss of small ground foraging native fauna and changes to burning and grazing regimes, many grassy woodland areas in eastern, southern and south-western Australia experienced an increase in woody vegetation in the mid to late 1800s. Clearing of this "regrowth" was a widespread activity in the late 1800s and early 1900s, partly to re-open areas for grazing and partly coincident with the expansion of cropping enterprises and the development of a railway system. This resulted in a misleading emphasis on trees when people tried to recall the nature of the original vegetation. To assess the pre-European vegetation as accurately as possible, the diaries and other records made by explorers and early surveyors need to be consulted.
There is absolutely no doubt that an increase in dryland salinity is closely linked to the loss of perennial native vegetation - but I believe it is the "overlooked understorey" which has undergone the most dramatic changes. That isn't to say that too many trees have not been removed from the landscape in some areas. However, in the majority of cases the reduction in soil health has been a function of declining organic carbon levels BELOW ground more so than above ground, due to the loss of perennial groundcover and the types of disturbance regimes which stimulate soil forming processes. The consequent reductions in root biomass, soil organic matter and surface litter on our agricultural land can be linked to many degradation processes including soil structural decline, nutrient decline, water repellence and increased soil acidity and sodicity.
Let's put the other land degradation issues to one side for the moment and focus on the salinisation process. There are two important pieces of information provided in the publication entitled "Assessing the causes, impacts, costs and management of dryland salinity" by Lin Martin and Jenni Metcalfe (LWRRDC Occasional Paper 20/98), which are worth repeating here:-
In a healthy catchment, salt is slowly leached downward and stored below the root zone.
In some dryland areas - especially those with winter rainfall patterns - recharge may have increased up to 100 times since clearing [of native vegetation] and the introduction of [annual] crops or pastures.
In other words we need a little bit of water going into deep drainage but not too much. If too much goes down it will come back up - with salt! Let's go through that again. In order to reverse the salinisation process, water has to be flushed downwards in the soil profile to take salts below the root zone, while at the same time not adding significantly to groundwater recharge. A fine juggling act indeed! This is only possible in the presence of a permanent groundcover of plants which enhance the infiltration of rainfall, have fibrous root systems with a high water holding capacity but low water use (thus holding water in the root zone for both plants and soil biota) and which facilitate the very slow percolation of some rainfall to groundwater.
You guessed it! Perennial native grasses fit these requirements perfectly, especially the tussock species which once inhabited many of the areas of Australia now plagued by accelerating dryland salinity. When grazed appropriately, a diverse stand of native tussock grasses (such as kangaroo grass, Themeda australis), inter-tussock grasses (such as microlaena, elymus) and forbs (such as native legumes, wild geranium, native plantain and wildflowers) can provide reliable year-round production and, if required, form an excellent base for direct-seeded grain or fodder crops. Crop production undertaken in conjunction with native groundcover has been shown to enhance, rather than compromise, both the crop and the grassland.
I'm not ignoring the ecosystem function of trees. Apart from the areas which are natural grasslands, up to 30% cover of trees and shrubs in the landscape can be extremely beneficial both ecologically and economically. Scattered trees may also help contain recharge. However, recent research has shown that plantation tree-lots, widely promoted as bandaids to utilise saline discharge water, often use the fresher water from the top 1-3 m of the soil, leading to an upward movement of the saltier groundwater from below, and slowed tree growth rates. That is, the movement of groundwater upwards becomes self-defeating. If you're relying on trees alone to solve your salinity problems, you'll be waiting a long time.
It surprises many people to discover that both root mass and microbial biomass can be much higher in a healthy perennial grassland soil than in a healthy sclerophyll forest soil. For a grassland to be healthy, however, requires much more than just the presence of grassland species. The biomass of the tops and the roots of grasses are roughly equal, forming a mirror image (Fig.1). Short grasses have short roots (Fig.1, LHS). Vigorous grasses have vigorous roots (Fig.1, RHS). The energy for root growth and metabolism can only come from sunlight trapped by the leaves during photosynthesis. A small leaf area cannot possibly support a large root system.
Fig. 1. The biomass of the roots and the tops of grasses are roughly equal, forming a mirror image. Short grasses (LHS) have small root systems.
In addition to increased soil water holding capacity and reduced groundwater recharge, large, fibrous root systems provide a multiplicity of other benefits including erosion control, soil aeration, habitat, and a continuous food supply for soil biota. The compromised root system of overgrazed or overrested plants cannot perform those functions very well. If there are no permanent roots in the soil at all, a whole suite of soil degradation problems will ensue, including soil structural decline and nutrient loss, and of course, in areas prone to salinisation, dryland salinity.
When perennial grasses are provided with an appropriate rest period (Fig. 2, RHS) and then defoliated in a single grazing event (using high stock densities such as in pulsed grazing), a large proportion of roots cease respiring and die in order to equalise the top and root biomass. These "pruned roots" provide an extremely important source of organic matter which improves the physical, chemical and biological attributes of the soil, including soil water holding capacity (more on this in Part III). The "pulsed" root pruning effect (Fig. 2, LHS) is therefore regenerative rather than degenerative. In the context of controlling recharge, the large volume of fibrous roots added to the soil system with each grazing pulse is invaluable (Fig. 2, LHS). This is a difficult concept for many people to grasp, because conventionally grazed grasses and annual grasses have shallow root systems which allow excessive leakage of rainfall to groundwater.
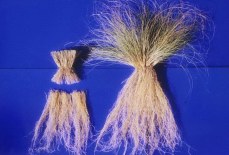
Fig. 2. When desirable grasses are rested (RHS) and then rapidly defoliated through pulsed grazing, the roots are effectively "pruned" within a few hours to equalise the biomass (LHS). The root pruning effect (LHS) is regenerative rather than degenerative.
It is fundamentally important that perennial grasses be rested prior to the next graze, to rebuild new root systems. If conditions are favourable, leaf regrowth will begin within hours of removal. However, re-grazing in the sensitive early stages will severely deplete plant reserves, resulting in either death or the formation of a steady-state type of equilibrium, where both tops and roots remain restricted in size, such as is found with mown turf and continuously grazed grassland.
Continuous grazing, that is, continuous root pruning, is a degenerative process. When pastures look like bowling greens, not only is the water cycle completely out of balance, but natural nutrient cycles can't function, plants are extremely vulnerable during droughts, and the low level of groundcover encourages weed invasion, erosion, and off-site impacts such as sedimentation and salinisation of dams and rivers. These degenerative processes also occur where the pasture base is annual, because groundcover and root biomass are not permanent. The severe nature of soil degradation, including salinisation, in the areas of southern and western Australia where annual crops, grasses and weeds have replaced perennial vegetation, bears witness to this. When there is no groundcover in the landscape at all (e.g. bare crop fallows) ………. need I go on? A special message for those croppers who have neither perennial groundcover nor livestock - think carefully about where you're headed in the next decade or so!
Like all ecosystem processes, salinisation is something we can control, provided we understand it. Reversing salinisation isn't easy, but we know it can be done. My key message is that bare ground, annual crops and pastures, and continuously grazed perennial pastures (in decreasing order) result in excessive groundwater recharge. High water use plants such as trees can be useful for lowering water tables, but the downside to high water-users is that they concentrate salt in the root zone. Let's start treating causes rather than applying bandaids to symptoms.
Plants with root systems which are "water-holders and slow drippers" are required. The inescapable solution for many of our agricultural areas is a permanent cover of fibrous-rooted, native perennial grasses, either as part of a diverse sward for grazing, or as a productive base for a multiplicity of other land use systems including cropping, horticulture, viticulture and alley-farmed silviculture. Because native grass roots hold water in the root zone, but the plants are themselves water-use efficient, other plants can be profitably grown in a native pasture base. Native trees and shrubs can also form a valuable component of the vegetation in areas which were originally grassy woodlands, for ecological, economic and aesthetic reasons, albeit ineffective for controlling the "root causes" of salinity.
Whatever your enterprise choice, think carefully about what's stopping YOU from controlling the salinisation process on your property. Remember, there are NO EXCUSES for bare ground outside the arid zone.
Part II Why the recharge-discharge model is fundamentally flawed
Part III Soil organic matter: past lessons for future learning
Part IV Understanding soil building processes
Part V Balanced soil, balanced water
Acknowledgments: The ideas presented here result from discussions with many people. In particular, I'd like to thank Darryl Cluff for helping me overcome my blinkered view of the nature of the pre-European vegetation and for supplying valuable reference materials. Special thanks also to Colin Seis who has been involved in salinity investigations on his own property "Winona" for well over a decade, and brought my attention to the "sponge-like" effect of C4 native grass roots in the soil. Fundamental to my view of ecosystem processes has been the visionary thinking of Allan Savory.